mirror of
https://git.tartarus.org/simon/putty.git
synced 2025-01-24 16:52:24 +00:00
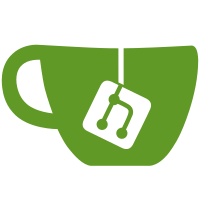
The number of people has been steadily increasing who read our source code with an editor that thinks tab stops are 4 spaces apart, as opposed to the traditional tty-derived 8 that the PuTTY code expects. So I've been wondering for ages about just fixing it, and switching to a spaces-only policy throughout the code. And I recently found out about 'git blame -w', which should make this change not too disruptive for the purposes of source-control archaeology; so perhaps now is the time. While I'm at it, I've also taken the opportunity to remove all the trailing spaces from source lines (on the basis that git dislikes them, and is the only thing that seems to have a strong opinion one way or the other). Apologies to anyone downstream of this code who has complicated patch sets to rebase past this change. I don't intend it to be needed again.
478 lines
15 KiB
C
478 lines
15 KiB
C
/*
|
|
* Prime generation.
|
|
*/
|
|
|
|
#include <assert.h>
|
|
#include "ssh.h"
|
|
#include "mpint.h"
|
|
|
|
/*
|
|
* This prime generation algorithm is pretty much cribbed from
|
|
* OpenSSL. The algorithm is:
|
|
*
|
|
* - invent a B-bit random number and ensure the top and bottom
|
|
* bits are set (so it's definitely B-bit, and it's definitely
|
|
* odd)
|
|
*
|
|
* - see if it's coprime to all primes below 2^16; increment it by
|
|
* two until it is (this shouldn't take long in general)
|
|
*
|
|
* - perform the Miller-Rabin primality test enough times to
|
|
* ensure the probability of it being composite is 2^-80 or
|
|
* less
|
|
*
|
|
* - go back to square one if any M-R test fails.
|
|
*/
|
|
|
|
/*
|
|
* The Miller-Rabin primality test is an extension to the Fermat
|
|
* test. The Fermat test just checks that a^(p-1) == 1 mod p; this
|
|
* is vulnerable to Carmichael numbers. Miller-Rabin considers how
|
|
* that 1 is derived as well.
|
|
*
|
|
* Lemma: if a^2 == 1 (mod p), and p is prime, then either a == 1
|
|
* or a == -1 (mod p).
|
|
*
|
|
* Proof: p divides a^2-1, i.e. p divides (a+1)(a-1). Hence,
|
|
* since p is prime, either p divides (a+1) or p divides (a-1).
|
|
* But this is the same as saying that either a is congruent to
|
|
* -1 mod p or a is congruent to +1 mod p. []
|
|
*
|
|
* Comment: This fails when p is not prime. Consider p=mn, so
|
|
* that mn divides (a+1)(a-1). Now we could have m dividing (a+1)
|
|
* and n dividing (a-1), without the whole of mn dividing either.
|
|
* For example, consider a=10 and p=99. 99 = 9 * 11; 9 divides
|
|
* 10-1 and 11 divides 10+1, so a^2 is congruent to 1 mod p
|
|
* without a having to be congruent to either 1 or -1.
|
|
*
|
|
* So the Miller-Rabin test, as well as considering a^(p-1),
|
|
* considers a^((p-1)/2), a^((p-1)/4), and so on as far as it can
|
|
* go. In other words. we write p-1 as q * 2^k, with k as large as
|
|
* possible (i.e. q must be odd), and we consider the powers
|
|
*
|
|
* a^(q*2^0) a^(q*2^1) ... a^(q*2^(k-1)) a^(q*2^k)
|
|
* i.e. a^((n-1)/2^k) a^((n-1)/2^(k-1)) ... a^((n-1)/2) a^(n-1)
|
|
*
|
|
* If p is to be prime, the last of these must be 1. Therefore, by
|
|
* the above lemma, the one before it must be either 1 or -1. And
|
|
* _if_ it's 1, then the one before that must be either 1 or -1,
|
|
* and so on ... In other words, we expect to see a trailing chain
|
|
* of 1s preceded by a -1. (If we're unlucky, our trailing chain of
|
|
* 1s will be as long as the list so we'll never get to see what
|
|
* lies before it. This doesn't count as a test failure because it
|
|
* hasn't _proved_ that p is not prime.)
|
|
*
|
|
* For example, consider a=2 and p=1729. 1729 is a Carmichael
|
|
* number: although it's not prime, it satisfies a^(p-1) == 1 mod p
|
|
* for any a coprime to it. So the Fermat test wouldn't have a
|
|
* problem with it at all, unless we happened to stumble on an a
|
|
* which had a common factor.
|
|
*
|
|
* So. 1729 - 1 equals 27 * 2^6. So we look at
|
|
*
|
|
* 2^27 mod 1729 == 645
|
|
* 2^108 mod 1729 == 1065
|
|
* 2^216 mod 1729 == 1
|
|
* 2^432 mod 1729 == 1
|
|
* 2^864 mod 1729 == 1
|
|
* 2^1728 mod 1729 == 1
|
|
*
|
|
* We do have a trailing string of 1s, so the Fermat test would
|
|
* have been happy. But this trailing string of 1s is preceded by
|
|
* 1065; whereas if 1729 were prime, we'd expect to see it preceded
|
|
* by -1 (i.e. 1728.). Guards! Seize this impostor.
|
|
*
|
|
* (If we were unlucky, we might have tried a=16 instead of a=2;
|
|
* now 16^27 mod 1729 == 1, so we would have seen a long string of
|
|
* 1s and wouldn't have seen the thing _before_ the 1s. So, just
|
|
* like the Fermat test, for a given p there may well exist values
|
|
* of a which fail to show up its compositeness. So we try several,
|
|
* just like the Fermat test. The difference is that Miller-Rabin
|
|
* is not _in general_ fooled by Carmichael numbers.)
|
|
*
|
|
* Put simply, then, the Miller-Rabin test requires us to:
|
|
*
|
|
* 1. write p-1 as q * 2^k, with q odd
|
|
* 2. compute z = (a^q) mod p.
|
|
* 3. report success if z == 1 or z == -1.
|
|
* 4. square z at most k-1 times, and report success if it becomes
|
|
* -1 at any point.
|
|
* 5. report failure otherwise.
|
|
*
|
|
* (We expect z to become -1 after at most k-1 squarings, because
|
|
* if it became -1 after k squarings then a^(p-1) would fail to be
|
|
* 1. And we don't need to investigate what happens after we see a
|
|
* -1, because we _know_ that -1 squared is 1 modulo anything at
|
|
* all, so after we've seen a -1 we can be sure of seeing nothing
|
|
* but 1s.)
|
|
*/
|
|
|
|
static unsigned short primes[6542]; /* # primes < 65536 */
|
|
#define NPRIMES (lenof(primes))
|
|
|
|
static void init_primes_array(void)
|
|
{
|
|
if (primes[0])
|
|
return; /* already done */
|
|
|
|
bool A[65536];
|
|
|
|
for (size_t i = 2; i < lenof(A); i++)
|
|
A[i] = true;
|
|
|
|
for (size_t i = 2; i < lenof(A); i++) {
|
|
if (!A[i])
|
|
continue;
|
|
for (size_t j = 2*i; j < lenof(A); j += i)
|
|
A[j] = false;
|
|
}
|
|
|
|
size_t pos = 0;
|
|
for (size_t i = 2; i < lenof(A); i++)
|
|
if (A[i])
|
|
primes[pos++] = i;
|
|
|
|
assert(pos == NPRIMES);
|
|
}
|
|
|
|
static unsigned short mp_mod_short(mp_int *x, unsigned short modulus)
|
|
{
|
|
/*
|
|
* This function lives here rather than in mpint.c partly because
|
|
* this is the only place it's needed, but mostly because it
|
|
* doesn't pay careful attention to constant running time, since
|
|
* as far as I can tell that's a lost cause for key generation
|
|
* anyway.
|
|
*/
|
|
unsigned accumulator = 0;
|
|
for (size_t i = mp_max_bytes(x); i-- > 0 ;) {
|
|
accumulator = 0x100 * accumulator + mp_get_byte(x, i);
|
|
accumulator %= modulus;
|
|
}
|
|
return accumulator;
|
|
}
|
|
|
|
/*
|
|
* Generate a prime. We can deal with various extra properties of
|
|
* the prime:
|
|
*
|
|
* - to speed up use in RSA, we can arrange to select a prime with
|
|
* the property (prime % modulus) != residue.
|
|
*
|
|
* - for use in DSA, we can arrange to select a prime which is one
|
|
* more than a multiple of a dirty great bignum. In this case
|
|
* `bits' gives the size of the factor by which we _multiply_
|
|
* that bignum, rather than the size of the whole number.
|
|
*
|
|
* - for the basically cosmetic purposes of generating keys of the
|
|
* length actually specified rather than off by one bit, we permit
|
|
* the caller to provide an unsigned integer 'firstbits' which will
|
|
* match the top few bits of the returned prime. (That is, there
|
|
* will exist some n such that (returnvalue >> n) == firstbits.) If
|
|
* 'firstbits' is not needed, specifying it to either 0 or 1 is
|
|
* an adequate no-op.
|
|
*/
|
|
mp_int *primegen(
|
|
int bits, int modulus, int residue, mp_int *factor,
|
|
int phase, progfn_t pfn, void *pfnparam, unsigned firstbits)
|
|
{
|
|
init_primes_array();
|
|
|
|
int progress = 0;
|
|
|
|
size_t fbsize = 0;
|
|
while (firstbits >> fbsize) /* work out how to align this */
|
|
fbsize++;
|
|
|
|
STARTOVER:
|
|
|
|
pfn(pfnparam, PROGFN_PROGRESS, phase, ++progress);
|
|
|
|
/*
|
|
* Generate a k-bit random number with top and bottom bits set.
|
|
* Alternatively, if `factor' is nonzero, generate a k-bit
|
|
* random number with the top bit set and the bottom bit clear,
|
|
* multiply it by `factor', and add one.
|
|
*/
|
|
mp_int *p = mp_power_2(bits - 1); /* ensure top bit is 1 */
|
|
mp_int *r = mp_random_bits(bits - 1);
|
|
mp_or_into(p, p, r);
|
|
mp_free(r);
|
|
mp_set_bit(p, 0, factor ? 0 : 1); /* set bottom bit appropriately */
|
|
|
|
for (size_t i = 0; i < fbsize; i++)
|
|
mp_set_bit(p, bits-fbsize + i, 1 & (firstbits >> i));
|
|
|
|
if (factor) {
|
|
mp_int *tmp = p;
|
|
p = mp_mul(tmp, factor);
|
|
mp_free(tmp);
|
|
assert(mp_get_bit(p, 0) == 0);
|
|
mp_set_bit(p, 0, 1);
|
|
}
|
|
|
|
/*
|
|
* We need to ensure this random number is coprime to the first
|
|
* few primes, by repeatedly adding either 2 or 2*factor to it
|
|
* until it is. To do this we make a list of (modulus, residue)
|
|
* pairs to avoid, and we also add to that list the extra pair our
|
|
* caller wants to avoid.
|
|
*/
|
|
|
|
/* List the moduli */
|
|
unsigned long moduli[NPRIMES + 1];
|
|
for (size_t i = 0; i < NPRIMES; i++)
|
|
moduli[i] = primes[i];
|
|
moduli[NPRIMES] = modulus;
|
|
|
|
/* Find the residue of our starting number mod each of them. Also
|
|
* set up the multipliers array which tells us how each one will
|
|
* change when we increment the number (which isn't just 1 if
|
|
* we're incrementing by multiples of factor). */
|
|
unsigned long residues[NPRIMES + 1], multipliers[NPRIMES + 1];
|
|
for (size_t i = 0; i < lenof(moduli); i++) {
|
|
residues[i] = mp_mod_short(p, moduli[i]);
|
|
if (factor)
|
|
multipliers[i] = mp_mod_short(factor, moduli[i]);
|
|
else
|
|
multipliers[i] = 1;
|
|
}
|
|
|
|
/* Adjust the last entry so that it avoids a residue other than zero */
|
|
residues[NPRIMES] = (residues[NPRIMES] + modulus - residue) % modulus;
|
|
|
|
/*
|
|
* Now loop until no residue in that list is zero, to find a
|
|
* sensible increment. We maintain the increment in an ordinary
|
|
* integer, so if it gets too big, we'll have to give up and go
|
|
* back to making up a fresh random large integer.
|
|
*/
|
|
unsigned delta = 0;
|
|
while (1) {
|
|
for (size_t i = 0; i < lenof(moduli); i++)
|
|
if (!((residues[i] + delta * multipliers[i]) % moduli[i]))
|
|
goto found_a_zero;
|
|
|
|
/* If we didn't exit that loop by goto, we've got our candidate. */
|
|
break;
|
|
|
|
found_a_zero:
|
|
delta += 2;
|
|
if (delta > 65536) {
|
|
mp_free(p);
|
|
goto STARTOVER;
|
|
}
|
|
}
|
|
|
|
/*
|
|
* Having found a plausible increment, actually add it on.
|
|
*/
|
|
if (factor) {
|
|
mp_int *d = mp_from_integer(delta);
|
|
mp_int *df = mp_mul(d, factor);
|
|
mp_add_into(p, p, df);
|
|
mp_free(d);
|
|
mp_free(df);
|
|
} else {
|
|
mp_add_integer_into(p, p, delta);
|
|
}
|
|
|
|
/*
|
|
* Now apply the Miller-Rabin primality test a few times. First
|
|
* work out how many checks are needed.
|
|
*/
|
|
unsigned checks =
|
|
bits >= 1300 ? 2 : bits >= 850 ? 3 : bits >= 650 ? 4 :
|
|
bits >= 550 ? 5 : bits >= 450 ? 6 : bits >= 400 ? 7 :
|
|
bits >= 350 ? 8 : bits >= 300 ? 9 : bits >= 250 ? 12 :
|
|
bits >= 200 ? 15 : bits >= 150 ? 18 : 27;
|
|
|
|
/*
|
|
* Next, write p-1 as q*2^k.
|
|
*/
|
|
size_t k;
|
|
for (k = 0; mp_get_bit(p, k) == !k; k++)
|
|
continue; /* find first 1 bit in p-1 */
|
|
mp_int *q = mp_rshift_safe(p, k);
|
|
|
|
/*
|
|
* Set up stuff for the Miller-Rabin checks.
|
|
*/
|
|
mp_int *two = mp_from_integer(2);
|
|
mp_int *pm1 = mp_copy(p);
|
|
mp_sub_integer_into(pm1, pm1, 1);
|
|
MontyContext *mc = monty_new(p);
|
|
mp_int *m_pm1 = monty_import(mc, pm1);
|
|
|
|
bool known_bad = false;
|
|
|
|
/*
|
|
* Now, for each check ...
|
|
*/
|
|
for (unsigned check = 0; check < checks && !known_bad; check++) {
|
|
/*
|
|
* Invent a random number between 1 and p-1.
|
|
*/
|
|
mp_int *w = mp_random_in_range(two, pm1);
|
|
monty_import_into(mc, w, w);
|
|
|
|
pfn(pfnparam, PROGFN_PROGRESS, phase, ++progress);
|
|
|
|
/*
|
|
* Compute w^q mod p.
|
|
*/
|
|
mp_int *wqp = monty_pow(mc, w, q);
|
|
mp_free(w);
|
|
|
|
/*
|
|
* See if this is 1, or if it is -1, or if it becomes -1
|
|
* when squared at most k-1 times.
|
|
*/
|
|
bool passed = false;
|
|
|
|
if (mp_cmp_eq(wqp, monty_identity(mc)) || mp_cmp_eq(wqp, m_pm1)) {
|
|
passed = true;
|
|
} else {
|
|
for (size_t i = 0; i < k - 1; i++) {
|
|
monty_mul_into(mc, wqp, wqp, wqp);
|
|
if (mp_cmp_eq(wqp, m_pm1)) {
|
|
passed = true;
|
|
break;
|
|
}
|
|
}
|
|
}
|
|
|
|
if (!passed)
|
|
known_bad = true;
|
|
|
|
mp_free(wqp);
|
|
}
|
|
|
|
mp_free(q);
|
|
mp_free(two);
|
|
mp_free(pm1);
|
|
monty_free(mc);
|
|
mp_free(m_pm1);
|
|
|
|
if (known_bad) {
|
|
mp_free(p);
|
|
goto STARTOVER;
|
|
}
|
|
|
|
/*
|
|
* We have a prime!
|
|
*/
|
|
return p;
|
|
}
|
|
|
|
/*
|
|
* Invent a pair of values suitable for use as 'firstbits' in the
|
|
* above function, such that their product is at least 2, and such
|
|
* that their difference is also at least min_separation.
|
|
*
|
|
* This is used for generating both RSA and DSA keys which have
|
|
* exactly the specified number of bits rather than one fewer - if you
|
|
* generate an a-bit and a b-bit number completely at random and
|
|
* multiply them together, you could end up with either an (ab-1)-bit
|
|
* number or an (ab)-bit number. The former happens log(2)*2-1 of the
|
|
* time (about 39%) and, though actually harmless, every time it
|
|
* occurs it has a non-zero probability of sparking a user email along
|
|
* the lines of 'Hey, I asked PuTTYgen for a 2048-bit key and I only
|
|
* got 2047 bits! Bug!'
|
|
*/
|
|
static inline unsigned firstbits_b_min(
|
|
unsigned a, unsigned lo, unsigned hi, unsigned min_separation)
|
|
{
|
|
/* To get a large enough product, b must be at least this much */
|
|
unsigned b_min = (2*lo*lo + a - 1) / a;
|
|
/* Now enforce a<b, optionally with minimum separation */
|
|
if (b_min < a + min_separation)
|
|
b_min = a + min_separation;
|
|
/* And cap at the upper limit */
|
|
if (b_min > hi)
|
|
b_min = hi;
|
|
return b_min;
|
|
}
|
|
|
|
void invent_firstbits(unsigned *one, unsigned *two, unsigned min_separation)
|
|
{
|
|
/*
|
|
* We'll pick 12 initial bits (number selected at random) for each
|
|
* prime, not counting the leading 1. So we want to return two
|
|
* values in the range [2^12,2^13) whose product is at least 2^25.
|
|
*
|
|
* Strategy: count up all the viable pairs, then select a random
|
|
* number in that range and use it to pick a pair.
|
|
*
|
|
* To keep things simple, we'll ensure a < b, and randomly swap
|
|
* them at the end.
|
|
*/
|
|
const unsigned lo = 1<<12, hi = 1<<13, minproduct = 2*lo*lo;
|
|
unsigned a, b;
|
|
|
|
/*
|
|
* Count up the number of prefixes of b that would be valid for
|
|
* each prefix of a.
|
|
*/
|
|
mp_int *total = mp_new(32);
|
|
for (a = lo; a < hi; a++) {
|
|
unsigned b_min = firstbits_b_min(a, lo, hi, min_separation);
|
|
mp_add_integer_into(total, total, hi - b_min);
|
|
}
|
|
|
|
/*
|
|
* Make up a random number in the range [0,2*total).
|
|
*/
|
|
mp_int *mlo = mp_from_integer(0), *mhi = mp_new(32);
|
|
mp_lshift_fixed_into(mhi, total, 1);
|
|
mp_int *randval = mp_random_in_range(mlo, mhi);
|
|
mp_free(mlo);
|
|
mp_free(mhi);
|
|
|
|
/*
|
|
* Use the low bit of randval as our swap indicator, leaving the
|
|
* rest of it in the range [0,total).
|
|
*/
|
|
unsigned swap = mp_get_bit(randval, 0);
|
|
mp_rshift_fixed_into(randval, randval, 1);
|
|
|
|
/*
|
|
* Now do the same counting loop again to make the actual choice.
|
|
*/
|
|
a = b = 0;
|
|
for (unsigned a_candidate = lo; a_candidate < hi; a_candidate++) {
|
|
unsigned b_min = firstbits_b_min(a_candidate, lo, hi, min_separation);
|
|
unsigned limit = hi - b_min;
|
|
|
|
unsigned b_candidate = b_min + mp_get_integer(randval);
|
|
unsigned use_it = 1 ^ mp_hs_integer(randval, limit);
|
|
a ^= (a ^ a_candidate) & -use_it;
|
|
b ^= (b ^ b_candidate) & -use_it;
|
|
|
|
mp_sub_integer_into(randval, randval, limit);
|
|
}
|
|
|
|
mp_free(randval);
|
|
mp_free(total);
|
|
|
|
/*
|
|
* Check everything came out right.
|
|
*/
|
|
assert(lo <= a);
|
|
assert(a < hi);
|
|
assert(lo <= b);
|
|
assert(b < hi);
|
|
assert(a * b >= minproduct);
|
|
assert(b >= a + min_separation);
|
|
|
|
/*
|
|
* Last-minute optional swap of a and b.
|
|
*/
|
|
unsigned diff = (a ^ b) & (-swap);
|
|
a ^= diff;
|
|
b ^= diff;
|
|
|
|
*one = a;
|
|
*two = b;
|
|
}
|